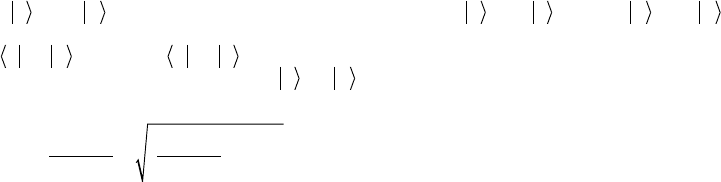
Slow Oscillation and Random Fluctuation in Quantum Dots: Can we Overcome? 385
12.5 Conventional optical study of QDs
Before we deal with the study of instabilities in the photo-luminescence of QDs, we shall present
the management of absorption and luminescence collected from a distribution of QDs with con-
ventional spectroscopic techniques, i.e. measurement involves many QDs having a distribution of
sizes. Recently, Liu et al. [27] , undertook placing CdSe QDs, 2.4 nm, in a photonic crystal con-
sisting of silica spheres with a diameter of 300 nm. The purpose is to match the photo-emission
to the forbidden gap of the photonic crystal for enhanced interaction. In one dimension, interac-
tion is enhanced by placing optically active transitions within the resonance of a Fabry–Perrot
interferometer.
The CdSe/ZnS core-shell QDs with 2.4 nm size were commercially available from Evident
Technologies Inc . To study the thiolation effect on the PL properties of QDs, several substrate sur-
faces, e.g. Si substrate, Au fi lm on Si, and fused silica, with and without sulphur were introduced,
and the root-mean-square (RMS) surface roughness of these samples was measured by a Veeco
Dimension 3100 atomic force microscope (AFM). Substrates were fi rst immersed in a solution of
5 mM (3-mercaptopropyl)-trimethoxysilane (MPTMS) in ethanol for 24 h, followed by a thiola-
tion process for introducing sulphur onto a substrate surface. To study the interaction among the
QDs themselves, low to high concentrations of QDs in toluene solution were used to deposit the
thin fi lm with QDs onto the above substrates via evaporation of the toluene solvent. 3D photonic
crystals of silica spheres were self-assembled onto the substrate via evaporation of the ethanol,
and the fi lm thickness of more than 15 layers was obtained. Thermal treatment was used to sta-
bilize the stop-band of the photonic crystals, involving annealing for 3 h in a quartz tube in the
range of 300 1000°C. A Cary 300 Bio UV-Visible spectrophotometer was used for the meas-
urements of the transmission spectrum at normal incidence. At this point one must recognize
that the experimental technique used for this study is no different from 50 years ago, except AFM
was used to characterize the structure and surface roughness.
To study the interaction between QDs and between QDs and the matrix, we start from a simple
but fundamental scheme. There are two types of shifts of the emission peak, a one-way shift, for
example caused by pressure, temperature, etc., and a two-way shift, one up-shift of the higher
peak and another down-shift of the lower peak caused by coupling. Let the electron wavefunc-
tions
1
and
2
represent two uncoupled states, with
E
o
11
1
and
HE
o
22
2
.
In
HEΨΨ
,
HH H
o
1
, where H
1
represents interaction. Defi ning a coupling term,
CH 1
1
2
and
CH
21
1
. The wavefunction Ψ can be expressed by a linear combina-
tion of the two uncoupled states,
Ψ ab12
, where a and b are two constants. The secular
determinant ( E E
1
)( E E
2
) C
2
0. The roots give the two shifted energy states:
E
EE EE
C
12 12
2
2
22
⎛
⎝
⎜
⎜
⎜
⎞
⎠
⎟
⎟
⎟
⎟
.
(12.5)
For two identical QDs, E
1
E
2
, so that E
E
1
C, and E
E
1
– C. Since electrons at the
upper state can relax to the lower state via phonons, the observed transition is from the lower
state to the ground states, assumed not coupled. In this process, the observed PL is red shifted
as observed. For QDs coupled to a matrix such as some sort of molecular surface complex, the
observed shift may go up or down depending on whether the interaction molecules are located
at lower energy or high energy, respectively. For convenience to the reader, we point out that
interaction with the matrix indicated that the molecule in question is located at a lower energy
because the PL is blue shifted. Before we show the measured shifts, let us point out the prob-
lem when a distribution of particle size is present. For two adjacent QDs with different sizes, the
full expression represented by Eq. 12.5 should be involved. Not only are the uncoupled spectra
broadened by distribution, the interaction, as shown in Eq. 12.5, has additional spreading. The
particle size distribution resulted in a PL linewidth being much more than the intrinsic thermal
broadening. Therefore the measured data we obtained do not land themselves to simple model-
ling; nevertheless, from an application point of view, what really matters is what we measured.
Furthermore, we did not know the details of compacting the QDs with dilution followed by sub-
sequent removing of the toluene solvent. We estimated an increase in packing density of perhaps
CH012-I046325.indd 385CH012-I046325.indd 385 6/24/2008 3:37:23 PM6/24/2008 3:37:23 PM