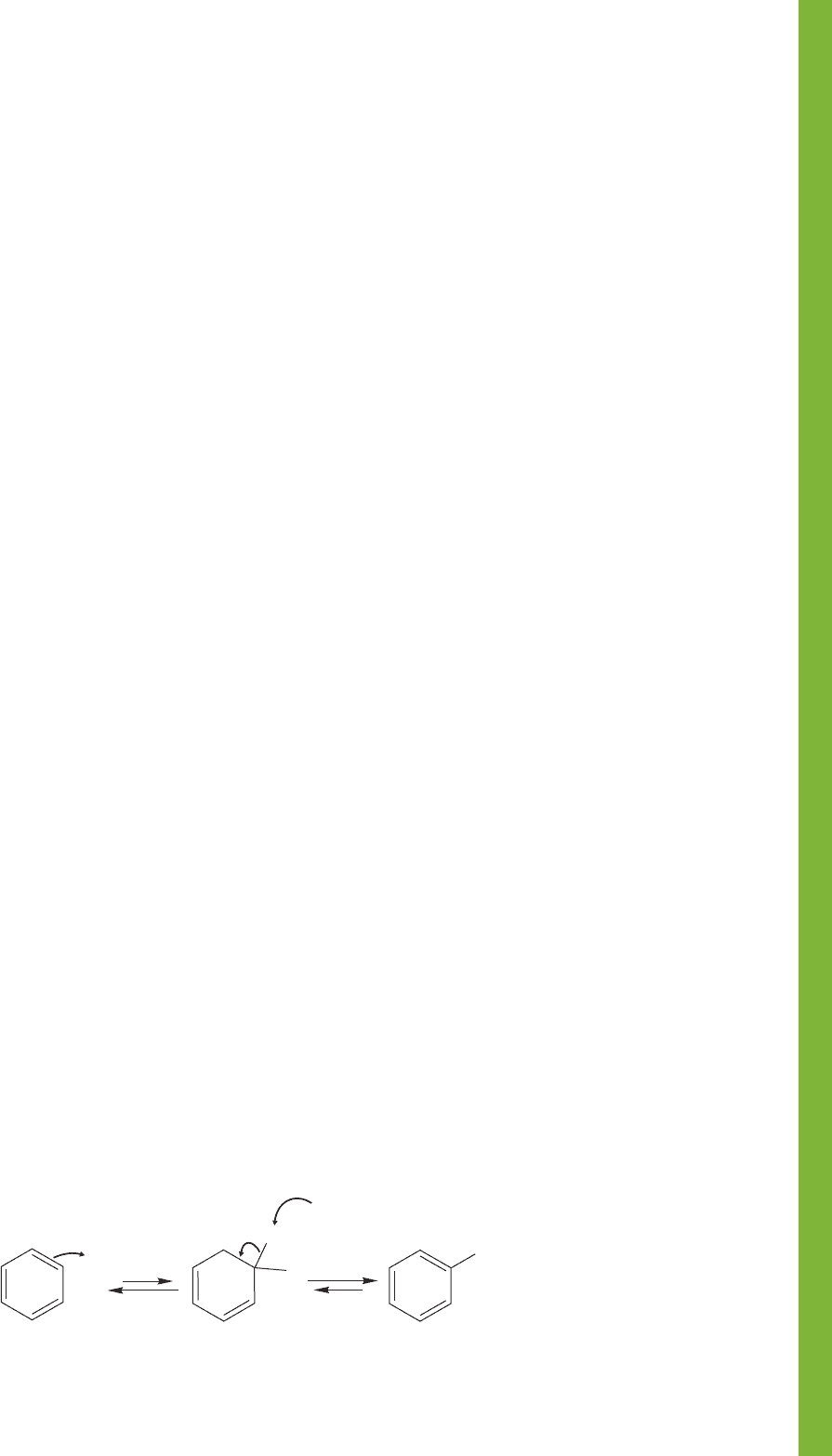
14.16 Summary 685
+
(+) (+)
BH
B
E
+
E
..
H
E
–
+
FIGURE 14.119 The general mechanism for electrophilic aromatic substitution.
Key Terms
acid chloride (p. 643)
acylium ion (p. 643)
benzyne (p. 681)
Chichibabin reaction (p. 676)
diazonium ion (p. 647)
electrophilic aromatic substitution
(p. 635)
Friedel–Crafts acylation (p. 643)
Friedel–Crafts alkylation (p. 639)
ipso attack (p. 677)
Meisenheimer complex (p. 675)
nucleophilic aromatic substitution (p. 675)
[n]paracyclophane (p. 628)
Sandmeyer reaction (p. 649)
superacid (p. 680)
Reactions, Mechanisms, and Tools
By far the most important reaction in this chapter is elec-
trophilic aromatic substitution by a variety of electrophiles (E
).
This reaction involves the initial formation of a resonance-
stabilized, but not aromatic, cyclohexadienyl cation.
Deprotonation regenerates an aromatic system (Fig. 14.119).
Nucleophilic aromatic substitution occurs only when
the ring carries substituents that can stabilize the negative
charge introduced through addition of a nucleophile.
Departure of a leaving group regenerates the aromatic system
(Fig. 14.100).
14.16 Summary
New Concepts
This chapter involves the chemistry of aromatic compounds
and is dominated by two fundamental interrelated notions.
First, it is simply difficult to disturb the aromatic sextet, and
second, once aromaticity is disrupted, it is easily regained. In
chemical terms, this means that reactions of benzene and
other simple aromatic compounds generally involve a highly
endothermic first step with formation of a high-energy inter-
mediate. In electrophilic aromatic substitution, the most com-
mon reaction of aromatic compounds, this intermediate is the
cyclohexadienyl cation. Because this intermediate lies far
above benzene in energy, the transition state leading to it is
also high in energy, and the reaction is difficult. Reactions of
the cyclohexadienyl cation that regenerate aromatic systems
are highly exothermic, and therefore have low activation ener-
gy barriers.
Further substitution reactions of an already substituted
benzene are controlled by the properties of the first sub-
stituent. Both resonance and inductive effects are involved, but
the resonance effect usually dominates. Many substituents can
stabilize an intermediate cyclohexadienyl cation through reso-
nance if substitution is in the ortho or para position. The extra
stabilization afforded by the substituent leads to relatively high
rates of reaction. Positively charged substituents generally sub-
stitute meta, because ortho or para substitution places two pos-
itive charges on adjacent positions. The introduction of a
second positive charge means that these substitutions are rela-
tively slow.
At low temperature, in sufficiently polar, but nonnucleo-
philic solvents, some carbocations are stable. Even under
these superacid conditions, rearrangements through hydride
or alkyl shifts to the most stable possible carbocation are
common.