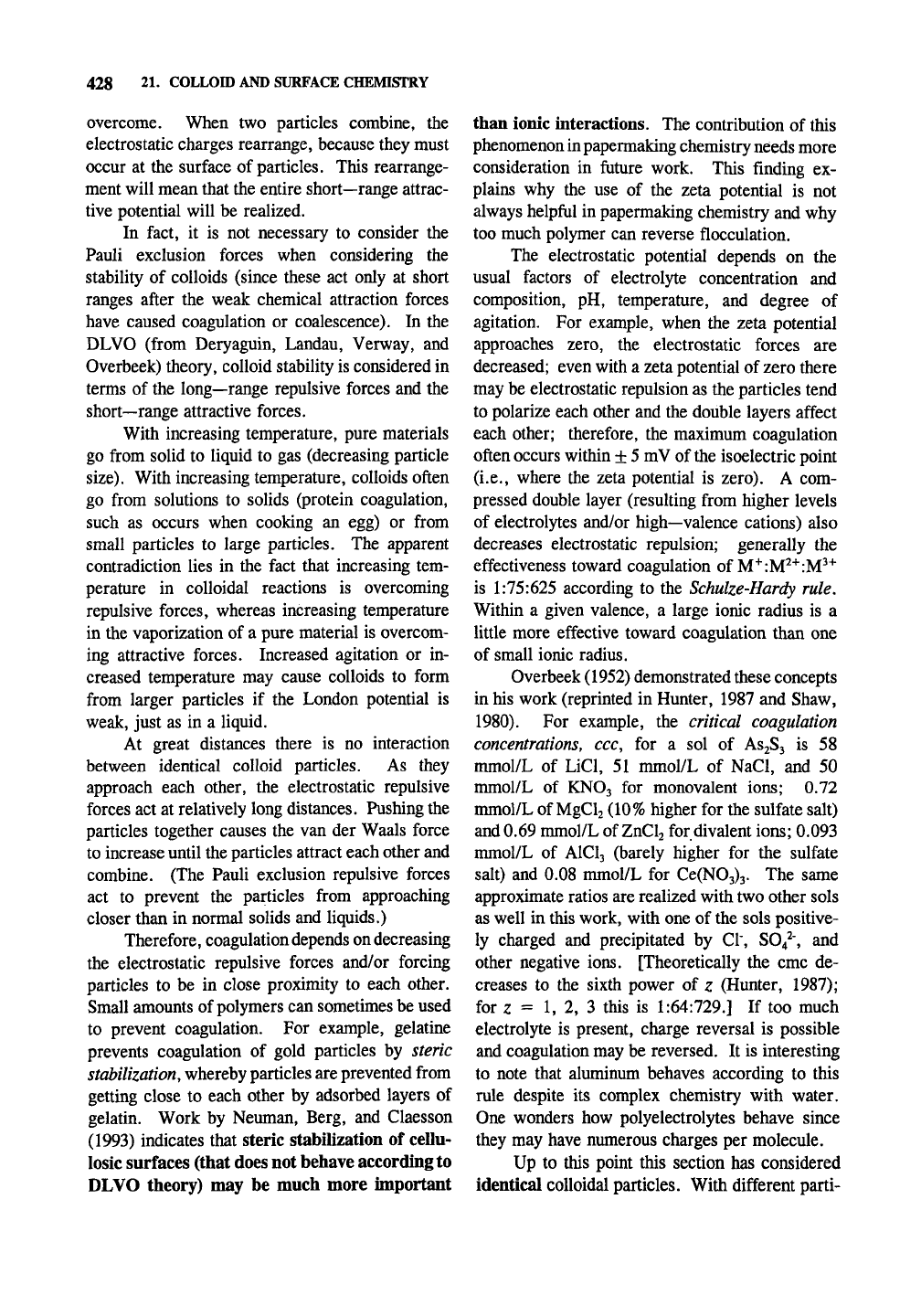
428 21. COLLOro AND SURFACE CHEMISTRY
overcome. When two particles combine, the
electrostatic charges rearrange, because they must
occur at the surface of particles. This rearrange-
ment will mean that the entire short—range attrac-
tive potential will be realized.
In fact, it is not necessary to consider the
Pauli exclusion forces when considering the
stability of colloids (since these act only at short
ranges after the weak chemical attraction forces
have caused coagulation or coalescence). In the
DLVO (from Deryaguin, Landau, Verway, and
Overbeek) theory, colloid stability is considered in
terms of the long—range repulsive forces and the
short—range attractive forces.
With increasing temperature, pure materials
go from solid to liquid to gas (decreasing particle
size).
With increasing temperature, colloids often
go from solutions to solids (protein coagulation,
such as occurs when cooking an egg) or from
small particles to large particles. The apparent
contradiction lies in the fact that increasing tem-
perature in colloidal reactions is overcoming
repulsive forces, whereas increasing temperature
in the vaporization of a pure material is overcom-
ing attractive forces. Increased agitation or in-
creased temperature may cause colloids to form
from larger particles if the London potential is
weak, just as in a liquid.
At great distances there is no interaction
between identical colloid particles. As they
approach each other, the electrostatic repulsive
forces act at relatively long distances. Pushing the
particles together causes the van der Waals force
to increase until the particles attract each other and
combine. (The Pauli exclusion repulsive forces
act to prevent the particles from approaching
closer than in normal solids and liquids.)
Therefore, coagulation
depends on
decreasing
the electrostatic repulsive forces and/or forcing
particles to be in close proximity to each other.
Small amounts of polymers can sometimes be used
to prevent coagulation. For example, gelatine
prevents coagulation of gold particles by steric
stabilization, whereby particles are prevented from
getting close to each other by adsorbed layers of
gelatin. Work by Neuman, Berg, and Claesson
(1993) indicates that steric stabilization of cellu-
losic surfaces (that does not behave according to
DLVO theory) may be much more important
than ionic interactions. The contribution of this
phenomenon in papermaking chemistry
needs
more
consideration in fixture work. This finding ex-
plains why the use of the zeta potential is not
always helpftil in papermaking chemistry and why
too much polymer can reverse flocculation.
The electrostatic potential depends on the
usual factors of electrolyte concentration and
composition, pH, temperature, and degree of
agitation. For example, when the zeta potential
approaches zero, the electrostatic forces are
decreased; even with a zeta potential of zero there
may be electrostatic repulsion as the particles tend
to polarize each other and the double layers affect
each other; therefore, the maximum coagulation
often occurs within + 5 mV of
the
isoelectric point
(i.e.,
where the zeta potential is zero). A com-
pressed double layer (resulting from higher levels
of electrolytes and/or high—valence cations) also
decreases electrostatic repulsion; generally the
effectiveness toward coagulation of M^rM^'^iM^'^
is
1:75:625
according to the Schulze-Hardy rule.
Within a given valence, a large ionic radius is a
little more effective toward coagulation than one
of small ionic radius.
Overbeek (1952) demonstrated these concepts
in his work (reprinted in Hunter, 1987 and Shaw,
1980).
For example, the critical coagulation
concentrationsy ccc, for a sol of AS2S3 is 58
mmol/L of LiCl, 51 mmol/L of NaCl, and 50
mmol/L of KNO3 for monovalent ions; 0.72
mmol/L of MgClj (10% higher for the sulfate salt)
and 0.69 mmol/L of
ZnCl2
for divalent ions; 0.093
mmol/L of AICI3 (barely higher for the sulfate
salt) and 0.08 mmol/L for Ce(N03)3. The same
approximate ratios are realized with two other sols
as well in this work, with one of the sols positive-
ly charged and precipitated by CI", 804^', and
other negative ions. [Theoretically the cmc de-
creases to the sixth power of z (Hunter, 1987);
for z = 1, 2, 3 this is
1:64:729.]
If too much
electrolyte is present, charge reversal is possible
and coagulation may be reversed. It is interesting
to note that aluminum behaves according to this
rule despite its complex chemistry with water.
One wonders how polyelectrolytes behave since
they may have numerous charges per molecule.
Up to this point this section has considered
identical colloidal particles. With different parti-