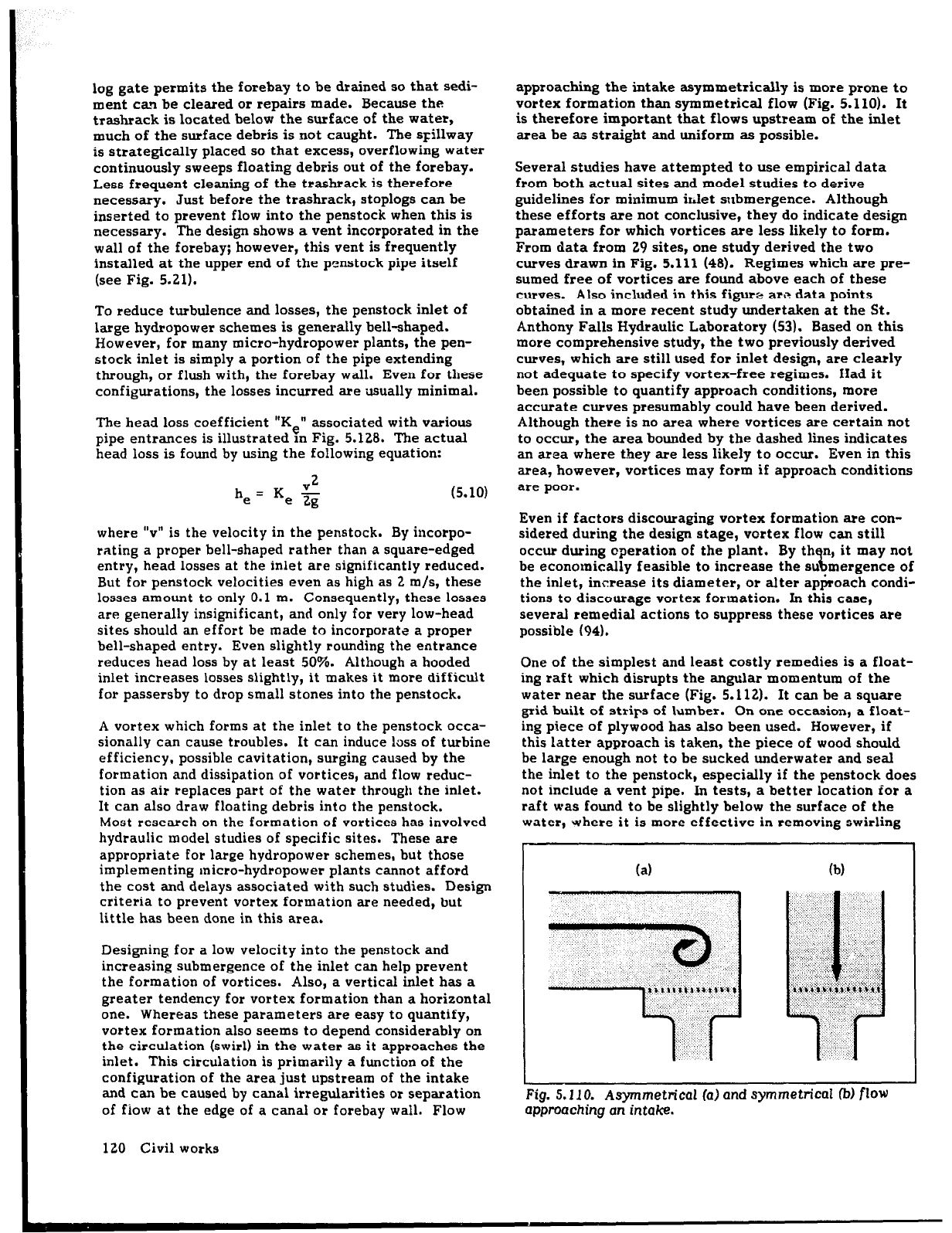
log gate permits the forebay to be drained so that sedi-
ment can be cleared or repairs made. Because the
trashrack is located below the surface of the water,
much of the surface debris is not caught. The spillway
is strategically placed so that excess, overflowing water
continuously sweeps floating debris out of the forebay.
Less frequent cleaning of the trashrack is therefore
necessary. Just before the trashrack, stoplogs can be
inserted to prevent flow into the penstock when this is
necessary.
The design shows a vent incorporated in the
wall of the forebay; however, this vent is frequently
installed at the upper end of the penstock pipe itself
(see Fig. 5.21).
To reduce turbulence and losses, the penstock inlet of
large hydropower schemes is generally bell-shaped.
However, for many micro-hydropower plants, the pen-
stock inlet is simply a portion of the pipe extending
through, or flush with, the forebay wall. Even for these
configurations, the losses incurred are *usually minimal.
The head loss coefficient “K ” associated with various
pipe entrances is illustrated ?n Fig. 5.128. The actual
head loss is found by using the following equation:
he= K
V2
e2g
(5.10)
where “v” is the velocity in the penstock. By incorpo-
rating a proper bell-shaped rather than a square-edged
entry, head losses at the inlet are significantly reduced.
But for penstock velocities even as high as 2 m/s, these
losses amount to only 0.1 m. Consequently, these losses
are generally insignificant, and only for very low-head
sites should an effort be made to incorporate a proper
bell-shaped entry. Even slightly rounding the entrance
reduces head loss by at least 50%. Although a hooded
inlet increases losses slightly, it makes it more difficult
for passersby to drop small stones into the penstock.
A vortex which forms at the inlet to the penstock occa-
sionally can cause troubles. It can induce loss of turbine
efficiency, possible cavitation, surging caused by the
formation and dissipation of vortices, and flow reduc-
tion as air replaces part of the water through the inlet.
It can also draw floating debris into the penstock.
Most research on the formation of vortices has involved
hydraulic model studies of specific sites. These are
appropriate for large hydropower schemes, but those
implementing micro-hydropower plants cannot afford
the cost and delays associated with such studies. Design
criteria to prevent vortex formation are needed, but
little has been done in this area.
Designing for a low velocity into the penstock and
increasing submergence of the inlet can help prevent
the formation of vortices. Also, a vertical inlet has a
greater tendency for vortex formation than a horizontal
one. Whereas these parameters are easy to quantify,
vortex formation also seems to depend considerably on
the circulation (swirl) in the water as it approaches the
inlet. This circulation is primarily a function of the
configuration of the area just upstream of the intake
and can be caused by canal irregularities or separation
of fiow at the edge of a canal or forebay wall. Flow
120 Civil works
approaching the intake asymmetrically is more prone to
vortex formation than symmetrical flow (Fig. 5.110). It
is therefore important that flows upstream of the inlet
area be as straight and uniform as possible.
Several studies have attempted to use empirical data
from both actual sites and model studies to derive
guidelines for minimum inlet submergence. Although
these efforts are not conclusive, they do indicate design
parameters for which vortices are less likely to form.
From data from 29 sites, one study derived the two
curves drawn in Fig. 5.111 (48). Regimes which are pre-
sumed free of vortices are found above each of these
curves. Also included in this figure are data points
obtained in a
more
recent study undertaken at the St.
Anthony Falls Hydraulic Laboratory (53). Based on this
more comprehensive study, the two previously derived
curves, which are still used for inlet design, are clearly
not adequate to specify vortex-free regimes. Had it
been possible to quantify approach conditions, more
accurate curves presumably could have been derived.
Although there is no area where vortices are certain not
to occur, the area bounded by the dashed lies indicates
an area where they are less likely to occur. Even in this
area, however, vortices may form if approach conditions
are poor.
Even if factors discouraging vortex formation are con-
sidered during the design stage, vortex flow can still
occur during operation of the plant. By th n, it may not
% be economically feasible to increase the su mergence of
the inlet, increase its diameter, or alter ap$roach condi-
tions to discourage vortex formation. In this case,
several remedial actions to suppress these vortices are
possible (94).
One of the simplest and least costly remedies is a float-
ing raft which disrupts the angular momentum of the
water near the surface (Fig. 5.112). It can be a square
grid built of strips of lumber. On one occasion, a float-
ing piece of plywood has also been used. However, if
this latter approach is taken, the piece of wood should
be large enough not to be sucked underwater and seal
the inlet to the penstock, especially if the penstock does
not include a vent pipe. In tests, a better location for a
raft was found to be slightly below the surface of the
water, Yrhere it is more effective in removing swirling
(a) 04
Fig.
5.110. Asymmetrical la)
and
symmetrical fb)
flow
approaching an intake.