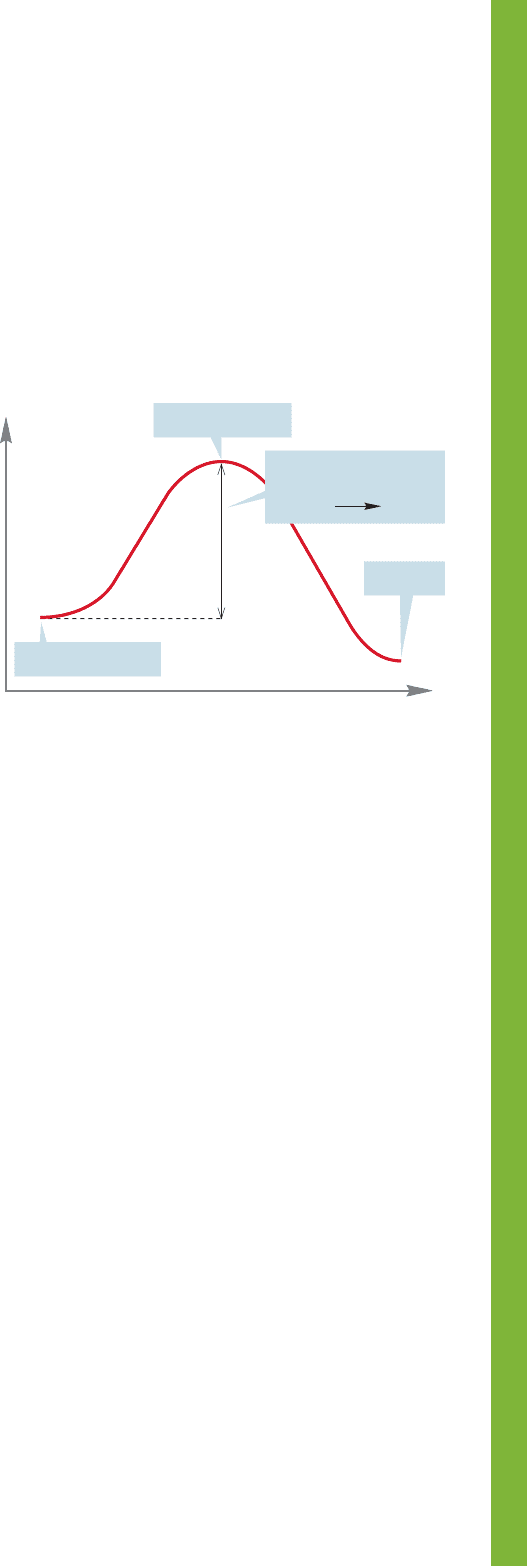
7.11 Summary 321
Another way to describe the reaction of Lewis bases with
Lewis acids is to use the graphic device showing the stabiliz-
ing interaction of a filled orbital (Lewis base) with an empty
orbital (Lewis acid) (Fig. 7.5). This idea can be generalized if
you recall that the strength of orbital interaction depends on
the energy difference between the orbitals involved. The closer
the orbitals are to each other in energy, the stronger is their
interaction and the greater is the resulting stabilization.
Therefore the strongest interactions between orbitals are likely
to be between the highest occupied molecular orbital
(HOMO) of one molecule (A, a Lewis base) and the lowest
unoccupied molecular orbital (LUMO) of the other (B,a
Lewis acid) (Fig. 7.7). This notion is used in this chapter to
explain the overwhelming preference for backside attack in
the S
N
2 reaction.
Stereochemical experiments are used here for the first
time to probe the details of reaction mechanisms. The
observation of inversion of configuration in the S
N
2
reaction shows that the incoming nucleophile must approach
the leaving group from the rear, the side opposite the
leaving group. Similarly, if the stereochemistry of the E2
reaction is monitored, it can be shown that there is a
strong preference for the anti (180°) E2 reaction in acyclic
molecules.
The curved arrow formalism is used extensively in this
chapter. This bookkeeping device is extremely useful in keeping
track of the pairs of electrons involved in the making and
breaking of bonds in a reaction.
The terms transition state (the structure representing
the high-energy point separating starting material and
products) and activation energy (the height of the transition
state above the starting material) appear several times in
this chapter. Chapter 8 will continue a detailed discussion
of these terms (Fig. 7.114).
Energy
Reaction progress
Transition state
Product
Starting material
Activation energy
for starting
material product
FIGURE 7.114 A typical Energy versus Reaction progress diagram.
Key Terms
anti elimination (p. 305)
E1cB reaction (p. 309)
E1 reaction (p. 298)
E2 reaction (p. 301)
elimination reaction (p. 298)
epoxide (p. 317)
Hofmann elimination (p. 308)
inversion of configuration (p. 269)
leaving group (p. 267)
nucleophilicity (p. 278)
oxirane (p. 317)
product-determining step (p. 294)
rate-determining step (p. 294)
reaction mechanism (p. 263)
regioselective reaction (p. 307)
retention of configuration (p. 269)
Saytzeff elimination (p. 300)
S
N
1 reaction (p. 289)
S
N
2 reaction (p. 268)
solvolysis reaction (p. 290)
substitution reaction (p. 262)
sulfonium ion (p. 313)
syn elimination (p. 305)
thionyl chloride (p. 284)
Williamson ether synthesis (p. 315)
Reactions, Mechanisms, and Tools
Four exceptionally important prototypal reactions are
introduced in this chapter: the S
N
1, S
N
2, E1, and E2
reactions. The E1 and S
N
1 reactions involve the same
carbocationic intermediate. Addition of a nucleophile com-
pletes the S
N
1 reaction; removal of a proton finishes the E1
(Fig. 7.70).
The S
N
2 reaction and the E2 reaction are also competitive
processes. In the S
N
2 reaction, a nucleophile attacks the sub-
strate at the rear of a carbon–leaving group bond. In the E2
reaction, it is the adjacent hydrogen that is removed along with
the leaving group (Fig. 7.74).
For primary substrates, it is the S
N
2 and E2 reactions that
compete. For tertiary substrates in polar solvents with weak
bases, it is the S
N
1 and E1 reactions that are important. If
strong bases are employed in nonionizing solvents, E2 reac-
tions are most important. For secondary substrates all four
reactions compete.
It is absolutely vital to have these four building block reac-
tion mechanisms under complete control.