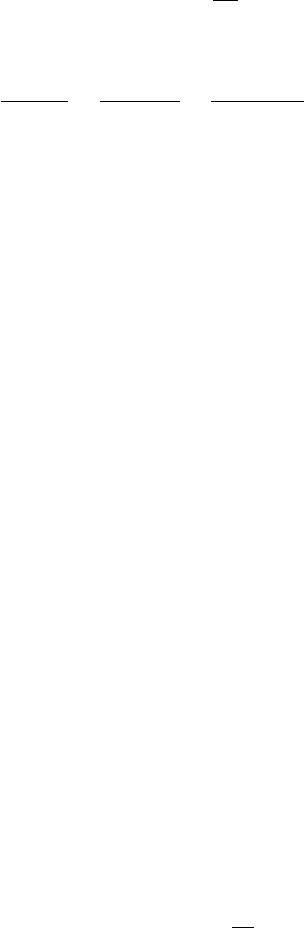
c06 JWPR067-Mench January 26, 2008 20:1 Char Count=
314 Polymer Electrolyte Fuel Cells
where n
d
has been shown to be ∼1.0–1.5. For water generation, we have Faraday’s law:
˙
n
H
2
O,gen
=
iA
2F
Comparing the two, we have
˙
n
H
2
O,drag
˙
n
H
2
O,gen
=
n
d
(iA/F)
iA/(2F )
=
(
1–1.5
)
× 2
1
= 2–3
Thus, water transport by electro-osmotic drag is always from the anode to cathode and is
two to three times greater than the water generation at the cathode for vapor-equilibrated
membranes! In terms of flooding at the cathode, the electro-osmotic drag to the cathode
results in more water than the electrochemical generation.
COMMENTS: In hydrogen PEFCs, much of the water driven to the cathode by electro-
osmotic drag is removed by back diffusion to the anode, especially in thin membranes. In
DAFCs with a liquid fuel solution, however, back diffusion does not occur and the cathode
flooding problem can be much more severe.
Temperature and Heat Flux Driven Flow A fourth mode of transport that has been shown
to drive water flux in the membrane is heat flux driven flow. As a general rule, water will
move through the membrane toward a colder location. This occurs in a freezing process
due to capillary forces [20, 21], and nonfreezing processes [22, 23]. The nonfrozen mode
of transport is poorly understood but is likely a result of the combined effects of capillary
pressure change with temperature and thermo-osmosis in membranes.
There may also be a phase change phenomena associated with the motion of water in
the membrane under a temperature gradient, since Bradean et al. [22] showed an exponential
relationship between liquid flux and the temperature gradient and heat flux, but the exact na-
ture of this is not fully understood. This mode of transport has not commonly been included
in the analysis of normal operation, since this effect is obscured by the net diffusive and
electro-osmotic drag transfer. Under startup or shutdown conditions, however, where larger
gradients in temperature can exist, the net water flux from this mode can be significant, and
has been exploited to passively drain the DM on shutdown to a frozen state [22].
Net Transport Coefficient The overall water transport through the membrane can be
written as the combination of the various modes of transport:
˙
n
H
2
O,
net,a−c
=
˙
n
H
2
O,
drag
+
˙
n
H
2
O,
diff
+
˙
n
H
2
O,
perm,gas
+
˙
n
H
2
O,
perm,cap
+
˙
n
H
2
O,
temp
(6.29)
The net drag coefficient α
d
is a parameter used to express the net drag of water from the
anode to the cathode and accounts for the total effect of the modes in Eq. (6.29):
˙
n
H
2
O,
net,a−c
= α
d
iA
F
(6.30)
The net drag coefficient represents the total transport of water to the cathode. In an ideal
situation for water management, α
d
would be uniformly −0.5 along the electrode. In this
case, the exact amount of water generated by reaction is exactly balanced and moved