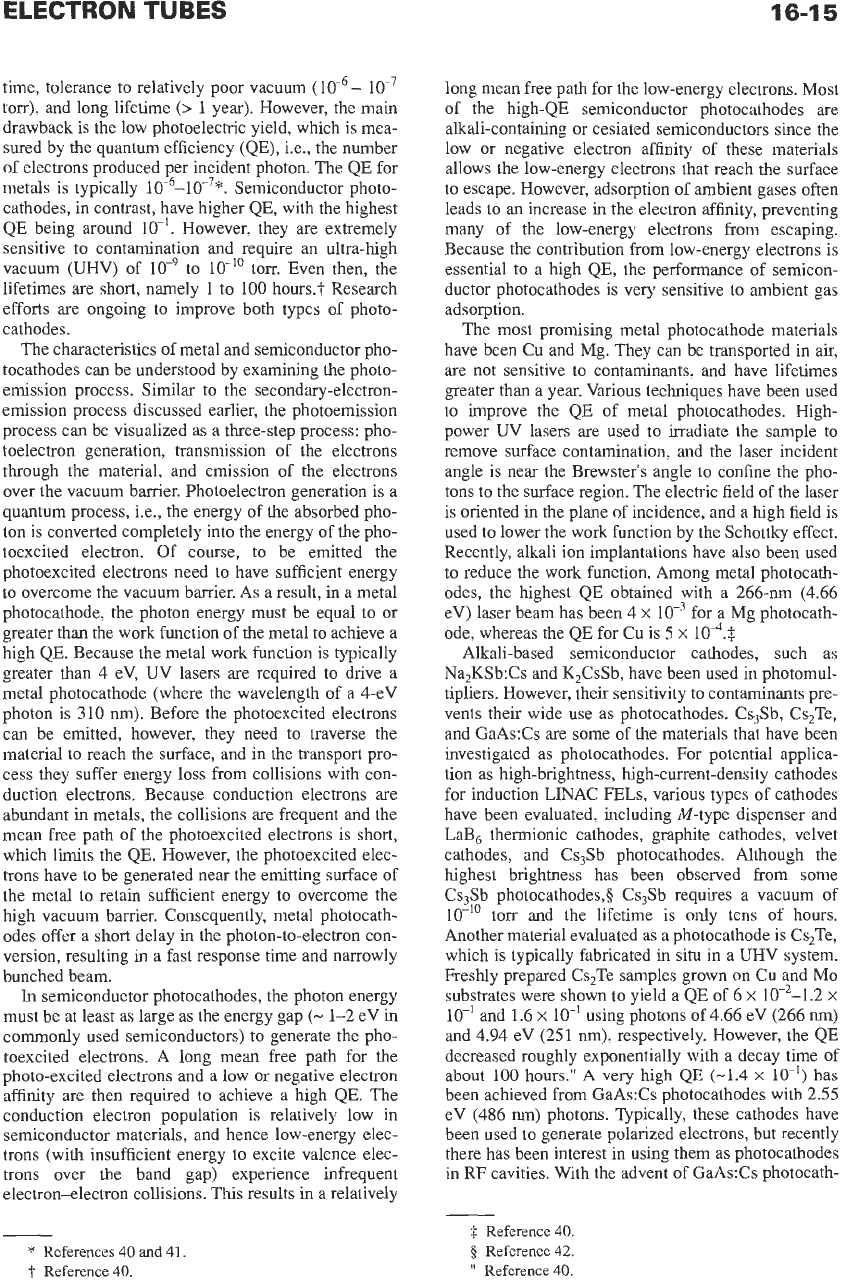
ELECTRON
TUBES
16-1
5
time, tolerance to relatively poor vacuum
-
10"
torr), and long lifetime
(>
1
year). However, the main
drawback is the low photoelectric yield, which is mea-
sured by the quantum efficiency (QE), i.e., the number
of electrons produced per incident photon. The QE for
metals is typically 10-6-10-7*. Semiconductor photo-
cathodes, in contrast, have higher QE, with the highest
QE being around
lo-'.
However, they are extremely
sensitive to contamination and require an ultra-high
vacuum (UHV) of to
lo-''
tom. Even then, the
lifetimes are short, namely
1
to
100
hours.? Research
efforts are ongoing to improve both types of photo-
cathodes.
The characteristics of metal and semiconductor pho-
tocathodes can be understood by examining the photo-
emission process. Similar to the secondary-electron-
emission process discussed earlier, the photoemission
process can be visualized as a three-step process: pho-
toelectron generation, transmission of the electrons
through the material, and emission of the electrons
over the vacuum barrier. Photoelectron generation is a
quantum process, i.e., the energy of the absorbed pho-
ton is converted completely into the energy of the pho-
toexcited electron. Of course, to be emitted the
photoexcited electrons need
to
have sufficient energy
to
overcome the vacuum barrier. As a result, in a metal
photocathode, the photon energy must be equal to or
greater than the work function of the metal to achieve a
high QE. Because the metal work function is typically
greater than 4 eV, UV lasers
are
required to drive a
metal photocathode (where the wavelength of a 4-eV
photon is
3
10
nm). Before the photoexcited electrons
can be emitted, however, they need to traverse the
material to reach the surface, and in the transport pro-
cess they suffer energy loss from collisions with con-
duction electrons. Because conduction electrons are
abundant in metals, the collisions are frequent and the
mean free path
of
the photoexcited electrons is short,
which limits the QE. However, the photoexcited elec-
trons have
to
be generated near the emitting surface of
the metal to retain sufficient energy
to
overcome the
high vacuum barrier. Consequently, metal photocath-
odes offer a short delay
in
the photon-to-electron con-
version, resulting
in
a fast response time and narrowly
bunched beam.
In
semiconductor photocathodes, the photon energy
must be at least as large as the energy gap
(-
1-2 eV
in
commonly used semiconductors) to generate the pho-
toexcited electrons. A long mean free path for the
photo-excited electrons and a
low
or negative electron
affinity are then required
to
achieve a high QE. The
conduction electron population is relatively low in
semiconductor materials, and hence low-energy elec-
trons (with insufficient energy
to
excite valence elec-
trons over the band gap) experience infrequent
electron-electron collisions.
This
results
in
a relatively
long mean free path for the low-energy electrons. Most
of
the high-QE semiconductor photocathodes
are
alkali-containing or cesiated semiconductors since the
low or negative electron affinity of these materials
allows the low-energy electrons that reach the surface
to escape. However, adsorption of ambient gases often
leads to an increase in the electron affinity, preventing
many of the low-energy electrons from escaping.
Because the contribution from low-energy electrons is
essential
to
a high QE, the performance of semicon-
ductor photocathodes is very sensitive
to
ambient gas
adsorption.
The most promising metal photocathode materials
have been Cu and Mg. They can be transported in air,
are not sensitive to contaminants, and have lifetimes
greater than a year. Various techniques have been used
to
improve the QE of metal photocathodes. High-
power UV lasers
are
used to irradiate the sample to
remove surface contamination, and the laser incident
angle is near the Brewster's angle
to
confine the pho-
tons to the surface region. The electric field of the laser
is oriented
in
the plane of incidence, and a high field is
used to lower the work function by the Schottky effect.
Recently, alkali ion implantations have also been used
to reduce the work function. Among metal photocath-
odes, the highest QE obtained with a 266-nm (4.66
eV) laser beam has been 4
x
for a Mg photocath-
ode, whereas the QE for Cu is
5
x
lo4.$
Alkali-based semiconductor cathodes, such as
Na,KSb:Cs and K,CsSb, have been used in photomul-
tipliers. However, their sensitivity to contaminants pre-
vents their wide use as photocathodes. Cs,Sb, Cs,Te,
and GaAs:Cs
are
some of the materials that have been
investigated as photocathodes. For potential applica-
tion as high-brightness, high-current-density cathodes
for induction LINAC
FELs,
various types of cathodes
have been evaluated, including M-type dispenser and
LaB, thermionic cathodes, graphite cathodes, velvet
cathodes, and Cs3Sb photocathodes. Although the
highest brightness has been observed from some
Cs,Sb photocathodes,§ Cs3Sb requires a vacuum of
lo-''
torr and the lifetime is only tens of hours.
Another material evaluated as a photocathode is Cs,Te,
which is typically fabricated
in
situ in a UHV system.
Freshly prepared Cs,Te samples grown on Cu and Mo
substrates were shown to yield a QE of 6
x
10-,-1.2
x
lo-'
and 1.6
x lo-'
using photons of 4.66 eV (266
nm)
and 4.94 eV (251 nm), respectively. However, the QE
decreased roughly exponentially with a decay time of
about 100 hours."
A
very high
QE
(-1.4
x
lo-')
has
been achieved from
GaAs:Cs
photocathodes with 2.55
eV (486
nm)
photons. Typically, these cathodes have
been used
to
generate polarized electrons, but recently
there has been interest in using them as photocathodes
in
RF
cavities. With the advent
of
GaAs:Cs photocath-
*
References 40
and
41
t
Reference 40.
$
Reference
40.
3
Reference
42.
"
Reference 40.