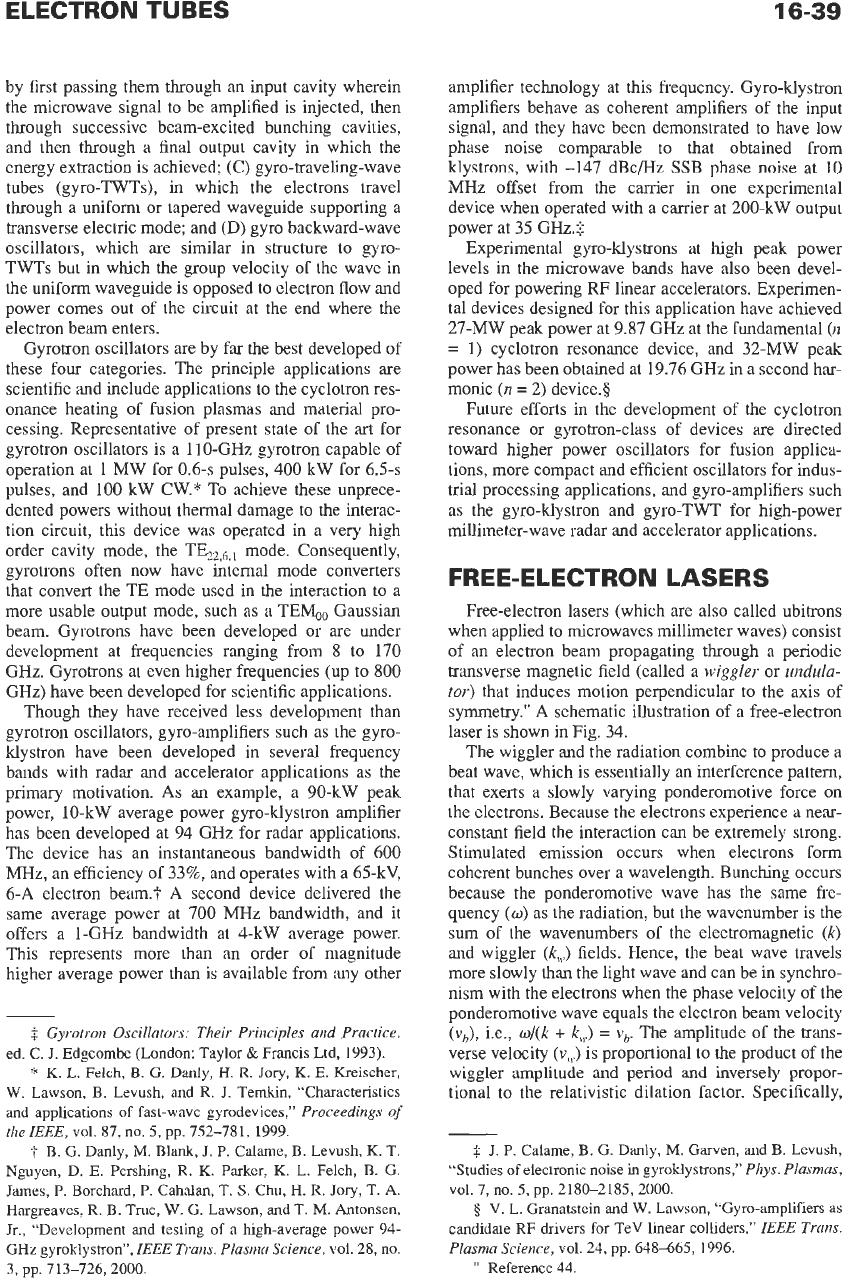
ELECTRON
TUBES
16-39
by first passing them through an input cavity wherein
the microwave signal to be amplified is injected, then
through successive beam-excited bunching cavities,
and then through a final output cavity in which the
energy extraction is achieved; (C) gyro-traveling-wave
tubes (gyro-TWTs),
in
which the electrons travel
through a uniform or tapered waveguide supporting a
transverse electric mode; and
(D)
gyro backward-wave
oscillators, which
are
similar in structure
to
gyro-
TWTs but in which the group velocity of the wave in
the uniform waveguide is opposed to electron flow and
power comes
out
of the circuit at the end where the
electron beam enters.
Gyrotron oscillators are by far the best developed of
these four categories. The principle applications are
scientific and include applications to the cyclotron res-
onance heating of fusion plasmas and material pro-
cessing. Representative of present state of the
art
for
gyrotron oscillators is a 110-GHz gyrotron capable of
operation at
1
MW for 0.6-s pulses, 400 kW for 6.5s
pulses, and
100
kW CW.* To achieve these unprece-
dented powers without thermal damage to the interac-
tion circuit, this device was operated in a very high
order cavity mode, the TE,,,,., mode. Consequently,
gyrotrons often now have internal mode converters
that convert the TE mode used in the interaction to a
more usable output mode, such as a TEW0 Gaussian
beam. Gyrotrons have been developed or are under
development at frequencies ranging from
8
to 170
GHz. Gyrotrons at even higher frequencies (up to
800
GHz) have been developed for scientific applications.
Though they have received less development than
gyrotroii oscillators, gyro-amplifiers such as the gyro-
klystron have been developed in several frequency
bands with radar and accelerator applications as the
primary motivation. As an example, a 90-kW peak
power, 10-kW average power gyro-klystron amplifier
has been developed at 94 GHz for radar applications.
The device has an instantaneous bandwidth of 600
MHz, an efficiency of 33%, and operates with a 65-kV,
6-A electron beam.?
A
second device delivered the
same average power at
700
MHz bandwidth, and it
offers a 1-GHz bandwidth at 4-kW average power.
This represents more than an order of magnitude
higher average power than is available from any other
$
Gyrotron Oscillators: Their Principles and Practice,
ed.
C.
J.
Edgcombe (London: Taylor
&
Francis Ltd, 1993).
*
K.
L.
Felch,
B.
G.
Danly,
H.
R.
Joy,
K.
E.
Kreischer,
W.
Lawson,
B.
Levush, and
R.
J.
Temkin, “Characteristics
and applications of fast-wave gyrodevices,”
Proceedings
of
the
IEEE,
vol. 87, no. 5, pp. 752-781, 1999.
t
B.
G. Danly,
M.
Blank,
J.
P.
Calame,
B.
Levush,
K.
T.
Nguyen, D.
E.
Pershing,
R.
K.
Parker,
K.
L. Felch,
B.
G.
James, P. Borchard, P. Cahalan, T.
S.
Chu, H.
R.
Jory,
T.
A.
Hargreaves,
R.
B.
True,
W.
G. Lawson,
and
T.
M.
Antonsen,
Jr., “Development and
testing
of
a high-average power 94-
GHz gyroklystron”,
IEEE
Trans. Plasmn Science,
vol.
28, no.
3, pp. 713-726,2000.
amplifier technology at this frequency. Gyro-klystron
amplifiers behave as coherent amplifiers of the input
signal, and they have been demonstrated to have low
phase noise comparable to that obtained from
klystrons, with -147 dBc/Hz
SSB
phase noise at
10
MHz offset from the carrier in one experimental
device when operated with a carrier at 200-kW output
power at 35 GHz.4
Experimental gyro-klystrons at high peak power
levels in the microwave bands have also been devel-
oped for powering RF linear accelerators. Experimen-
tal devices designed for this application have achieved
27-MW peak power at 9.87 GHz at the fundamental
(n
=
1)
cyclotron resonance device, and 32-MW peak
power has been obtained at 19.76 GHz in a second har-
monic
(n
=
2) device.§
Future efforts in the development
of
the cyclotron
resonance or gyrotron-class of devices are directed
toward higher power oscillators for fusion applica-
tions, more compact and efficient oscillators for indus-
trial processing applications, and gyro-amplifiers such
as the gyro-klystron and gyro-TWT for high-power
millimeter-wave radar and accelerator applications.
FREE-ELECTRON LASERS
Free-electron lasers (which are also called ubitrons
when applied to microwaves millimeter waves) consist
of an electron beam propagating through a periodic
transverse magnetic field (called a
wiggler
or
undula-
tor)
that induces motion perpendicular to the axis of
symmetry.” A schematic illustration of a free-electron
laser is shown in Fig. 34.
The wiggler and the radiation combine
to
produce a
beat wave, which is essentially an interference pattern,
that exerts a slowly varying ponderomotive force
on
the electrons. Because the electrons experience a near-
constant field the interaction can be extremely strong.
Stimulated emission occurs when electrons form
coherent bunches over a wavelength. Bunching occurs
because the ponderomotive wave has the same fre-
quency
(w)
as the radiation, but the wavenumber is the
sum of the wavenumbers of the electromagnetic
(k)
and wiggler
(k,)
fields. Hence, the beat wave travels
more slowly than the light wave and can be in synchro-
nism with the electrons when the phase velocity of the
ponderomotive wave equals the electron beam velocity
(vb),
i.e.,
w/(k
+
kw,)
=
vb
The amplitude of the trans-
verse velocity
(v,J
is proportional to the product of the
wiggler
amplitude
and period and inversely
propor-
tional to the relativistic dilation factor. Specifically,
$
J.
P.
Calame,
B.
G. Danly,
M.
Gwen, and
B.
Levush,
“Studies of electronic noise
in
gyroklystrons,”
Phys. Plasmas,
vol. 7, no. 5, pp. 2180-2185,2000.
5
V. L. Granatstein and
W.
Lawson, “Gyro-amplifiers as
candidate
RF
drivers for TeV linear colliders,”
IEEE
Trans.
Plasma Science,
vol.
24,
pp.
648-665, 1996.
”
Reference
44.