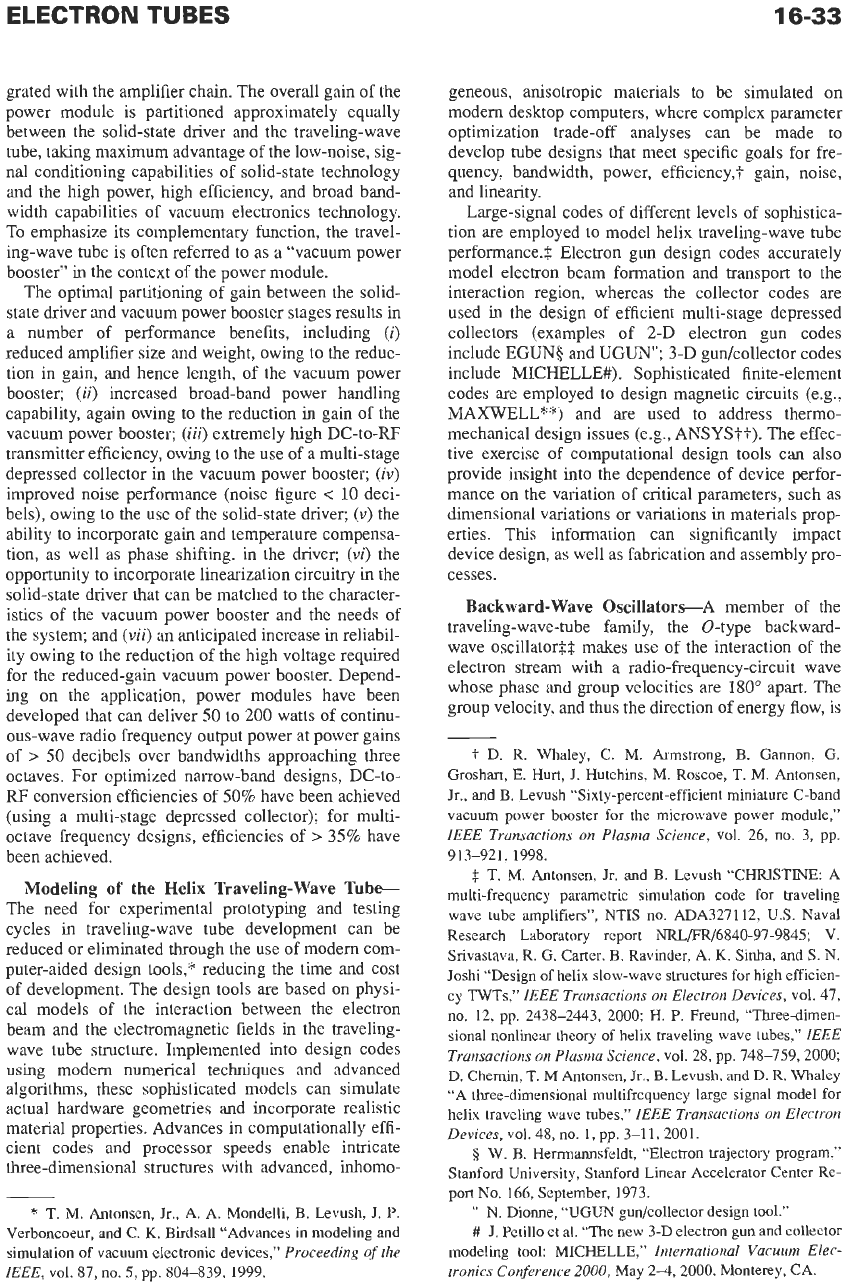
ELECTRON TUBES
16-33
grated with the amplifier chain. The overall gain of the
power module is partitioned approximately equally
between the solid-state driver and the traveling-wave
tube, taking maximum advantage of the low-noise, sig-
nal conditioning capabilities of solid-state technology
and the high power, high efficiency, and broad band-
width capabilities of vacuum electronics technology.
To emphasize its complementary function, the travel-
ing-wave tube is often referred
to
as a “vacuum power
booster” in the context of the power module.
The optimal partitioning of gain between the solid-
state driver and vacuum power booster stages results in
a
number of performance benefits, including
(i)
reduced amplifier size and weight, owing to
the
reduc-
tion
in
gain, and hence length, of the vacuum power
booster;
(ii)
increased broad-band power handling
capability, again owing
to
the reduction in gain of the
vacuum power booster;
(iii)
extremely high DC-to-RF
transmitter efficiency, owing to the use of a multi-stage
depressed collector in the vacuum power booster;
(iv)
improved noise performance (noise figure
c:
10
deci-
bels), owing
to
the use of the solid-state driver;
(v)
the
ability to incorporate gain and temperature compensa-
tion, as well as phase shifting, in the driver;
(vi)
the
opportunity to incorporate linearization circuitry in the
solid-state driver that can be matched to the character-
istics of the vacuum power booster and the needs of
the system; and
(vii)
an anticipated increase in reliabil-
ity owing to the reduction of the high voltage required
for the reduced-gain vacuum power booster. Depend-
ing
on
the application, power modules have been
developed that can deliver
50
to
200
watts of continu-
ous-wave radio frequency
output
power at power gains
of
>
50
decibels over bandwidths approaching three
octaves. For optimized narrow-band designs, DC-to-
RF
conversion efficiencies of
50%
have been achieved
(using a multi-stage depressed collector); for multi-
octave frequency designs, efficiencies of
>
35%
have
been achieved.
Modeling
of
the Helix Traveling-Wave
Tub+
The need for experimental prototyping and testing
cycles in traveling-wave tube development can be
reduced or eliminated through the use of modem com-
puter-aided design tools,* reducing the time and cost
of development. The design tools are based
on
physi-
cal models of the interaction between the electron
beam and the electromagnetic fields in the traveling-
wave tube structure. Implemented into design codes
using
modem
numerical
techniques
and advanced
algorithms, these sophisticated models can simulate
actual hardware geometries and incorporate realistic
material properties. Advances in computationally effi-
cient codes and processor speeds enable intricate
three-dimensional structures with advanced, inhomo-
*
T.
M.
Antonsen, Jr., A. A. Mondelli,
B.
Levush,
J.
P.
Verboncoeur, and C.
K.
Birdsall “Advances in modeling and
simulation of vacuum electronic devices,”
Proceeding of the
IEEE,
vol.
87,
no.
5,
pp. 806839,1999.
geneous, anisotropic materials to be simulated
on
modem desktop computers, where complex parameter
optimization trade-off analyses can be made to
develop tube designs that meet specific goals for fre-
quency, bandwidth, power, efficiency,? gain, noise,
and linearity.
Large-signal codes of different levels of sophistica-
tion
are
employed
to
model helix traveling-wave tube
performance.$ Electron gun design codes accurately
model electron beam formation and transport to the
interaction region, whereas the collector codes are
used in the design of efficient multi-stage depressed
collectors (examples of 2-D electron gun codes
include
EGUNC)
and
UGUN“;
3-D gun/collector codes
include MICHELLE#). Sophisticated finite-element
codes are employed to design magnetic circuits (e.g.,
MAXWELL**) and are used to address thermo-
mechanical design issues (e.g.,
ANSYSTt).
The effec-
tive exercise of computational design tools can also
provide insight into the dependence of device perfor-
mance
on
the variation of critical parameters, such as
dimensional variations or variations in materials prop-
erties.
This
information can significantly impact
device design,
as
well as fabrication and assembly pro-
cesses.
Backward-Wave Oscillators-A
member of the
traveling-wave-tube family, the 0-type backward-
wave oscillator$$ makes use of the interaction of the
electron stream with
a
radio-frequency-circuit wave
whose phase and group velocities
are
180”
apart. The
group velocity, and thus the direction of energy flow, is
t
D.
R.
Whaley, C. M. Armstrong, B. Gannon,
G.
Groshart,
E.
Hurt,
J.
Hutchins,
M.
Roscoe,
T.
M.
Antonsen,
Jr., and B. Levush “Sixty-percent-efficient miniature C-band
vacuum power booster for the microwave power module,”
IEEE Transactions on Plasma Science,
vol. 26, no. 3, pp.
913-921,1998.
f
T.
M.
Antonsen, Jr. and B. Levush “CHRISTINE: A
multi-frequency parametric simulation code for traveling
wave tube amplifiers”,
NTIS
no. ADA327112. U.S. Naval
Research Laboratory report NRL/FR/6840-97-9845; V.
Srivastava, R. G. Carter, B. Ravinder, A.
K.
Sinha, and
S.
N.
Joshi “Design of helix slow-wave structures for high efficien-
cy
TWTs,”
IEEE Transactions on Electron Devices,
vol. 41,
no. 12,
pp.
2438-2443,
2000;
H.
P.
Freund, “Three-dimen-
sional nonlinear theory
of
helix traveling wave tubes,”
IEEE
Transactions on Plasma Science,
vol.
28,
pp.
748-759,200&
D. Chemin,
T.
M
Antonsen,
Jr.,
B.
Levush, and
D.
R.
Whaley
“A three-dimensional multifrequency large signal model for
helix traveling wave tubes,”
IEEE Transactions on Electron
Devices,
vol. 48, no.
1,
pp. 3-1 1,2001.
Q
W.
B. Herrmannsfeldt, “Electron trajectory program.”
Stanford University, Stanford Linear Accelerator Center Re-
port
No.
166, September, 1973.
“
N.
Dionne,
“UGUN
gun/collector design tool.”
#
J.
Petillo et al. “The new 3-D electron gun and collector
modeling tool: MICHELLE,”
International Vacuum Elec-
tronics Conference
2000, May
2-4,
2000,
Monterey, CA.