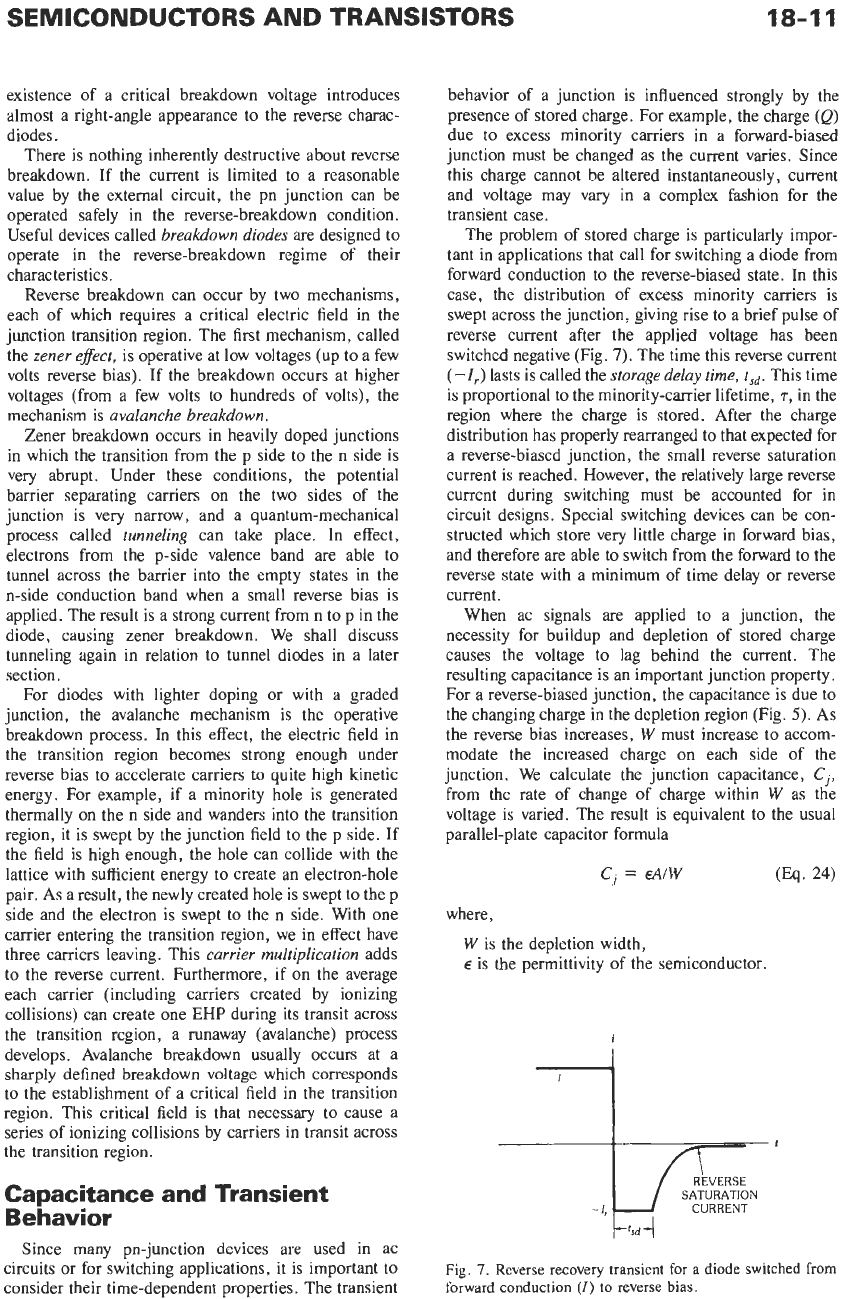
18-11
existence of a critical breakdown voltage introduces
almost a right-angle appearance to the reverse charac-
diodes.
There
is
nothing inherently destructive about reverse
breakdown. If the current is limited to a reasonable
value by the external circuit, the pn junction can be
operated safely in the reverse-breakdown condition.
Useful devices called
breakdown
diodes
are designed to
operate in the reverse-breakdown regime of their
characteristics.
Reverse breakdown can occur by two mechanisms,
each of which requires a critical electric field in the
junction transition region. The first mechanism, called
the
zener
effect,
is operative at low voltages (up to a few
volts reverse bias). If the breakdown occurs at higher
voltages (from a few volts to hundreds of volts), the
mechanism is
avalanche breakdown.
Zener breakdown occurs in heavily doped junctions
in which the transition from the p side to the n side is
very abrupt. Under these conditions, the potential
barrier separating carriers on the two sides of the
junction
is
very narrow, and a quantum-mechanical
process called
tunneling
can take place. In effect,
electrons from the p-side valence band are able to
tunnel across the barrier into the empty states in the
n-side conduction band when a small reverse bias is
applied. The result is a strong current from n to p in the
diode, causing zener breakdown. We shall discuss
tunneling again in relation to tunnel diodes in a later
section.
For diodes with lighter doping or with a graded
junction, the avalanche mechanism is the operative
breakdown process. In this effect, the electric field in
the transition region becomes strong enough under
reverse bias to accelerate carriers to quite high kinetic
energy. For example, if a minority hole is generated
thermally on the n side and wanders into the transition
region, it is swept by the junction field to the p side. If
the field is high enough, the hole can collide with the
lattice with sufficient energy to create an electron-hole
pair.
As
a result, the newly created hole is swept to the p
side and the electron
is
swept to the n side. With one
carrier entering the transition region, we in effect have
three carriers leaving. This
carrier multiplication
adds
to the reverse current. Furthermore, if on the average
each carrier (including carriers created by ionizing
collisions) can create one
EHP
during its transit across
the transition region, a runaway (avalanche) process
develops. Avalanche breakdown usually occurs at a
sharply
defined breakdown voltage which corresponds
to the establishment of a critical field in the transition
region. This critical field is that necessary to cause a
series
of
ionizing collisions by carriers in transit across
the transition region.
Capacitance and Transient
Behavior
Since many pn-junction devices are used in ac
circuits or for switching applications, it is important to
consider their time-dependent properties. The transient
behavior of a junction is influenced strongly by the
presence of stored charge. For example, the charge
(Q)
due to excess minority carriers in a forward-biased
junction must be changed as the current varies. Since
this charge cannot be altered instantaneously, current
and voltage may vary in a complex fashion for the
transient case.
The problem of stored charge is particularly impor-
tant in applications that call for switching a diode from
forward conduction to the reverse-biased state. In this
case, the distribution of excess minority carriers is
swept across the junction, giving rise to a brief pulse of
reverse current after the applied voltage has been
switched negative (Fig.
7).
The time this reverse current
(
-Zr)
lasts is called the
storage
deZay
time,
tsd.
This time
is proportional to the minority-carrier lifetime,
T,
in the
region where the charge is stored. After the charge
distribution has properly rearranged to that expected for
a reverse-biased junction, the small reverse saturation
current is reached. However, the relatively large reverse
current during switching must be accounted for in
circuit designs. Special switching devices can be con-
structed which store very little charge in forward bias,
and therefore are able to switch from the forward to the
reverse state with a minimum of time delay or reverse
current.
When ac signals are applied to a junction, the
necessity for buildup and depletion of stored charge
causes the voltage to lag behind the current. The
resulting capacitance is an important junction property.
For a reverse-biased junction, the capacitance is due to
the changing charge in the depletion region (Fig.
5).
As
the reverse bias increases,
W
must increase to accom-
modate the increased charge on each side of the
junction. We calculate the junction capacitance,
Cj,
from the rate of change of charge within
W
as the
voltage is varied. The result is equivalent to the usual
parallel-plate capacitor formula
ci
=
€A/w
(Eq.
24)
where,
W
is the depletion width,
E
is the permittivity of the semiconductor.
i
I,+
CURRENT
kf5d-I
Fig.
7.
Reverse
recovery transient for a
diode
switched from
forward
conduction
(I)
to reverse bias.