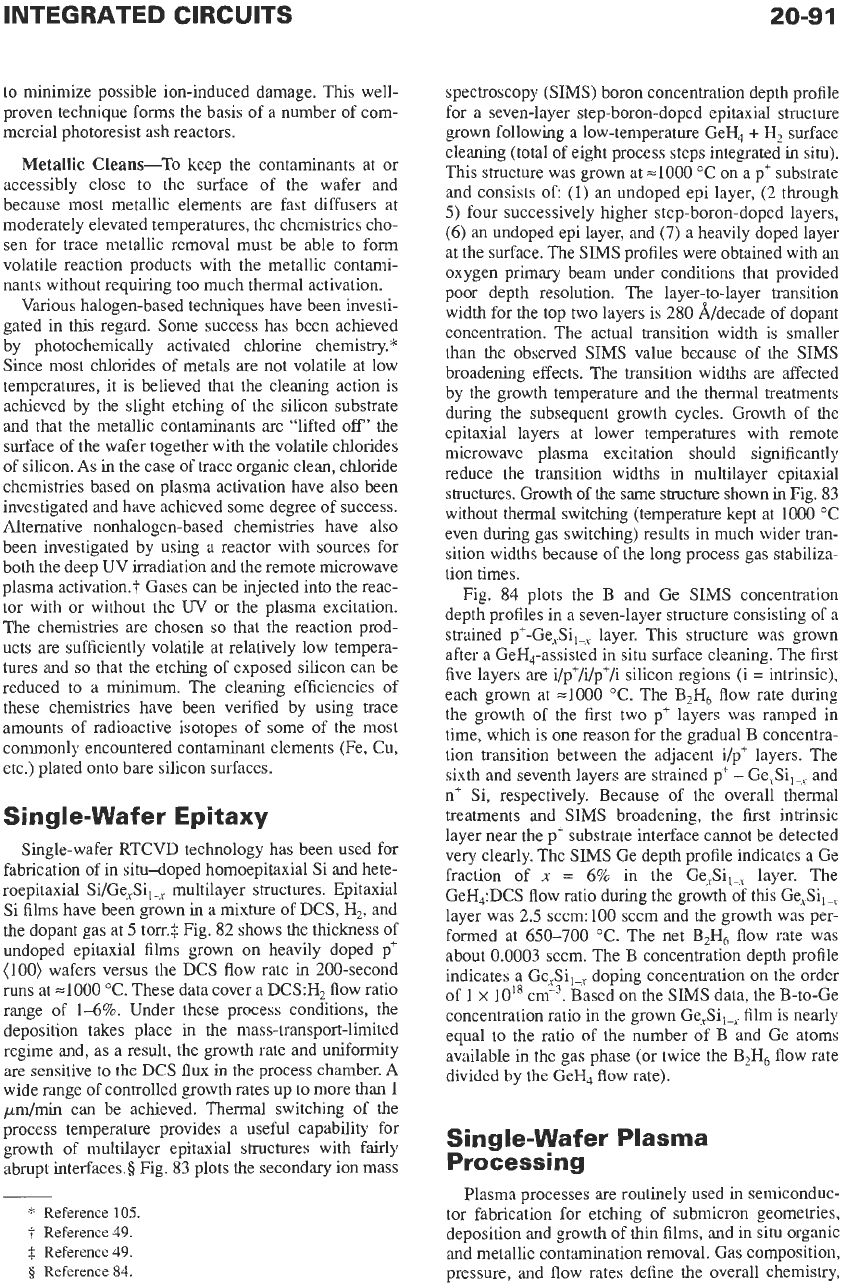
INTEGRATED CIRCUITS
20-91
to minimize possible ion-induced damage. This well-
proven technique forms the basis of a number of com-
mercial photoresist ash reactors.
Metallic
Cleans-To keep the contaminants at or
accessibly close to the surface of the wafer and
because most metallic elements are fast diffusers at
moderately elevated temperatures, the chemistries cho-
sen for trace metallic removal must be able to form
volatile reaction products with
the
metallic contami-
nants without requiring too much thermal activation.
Various halogen-based techniques have been investi-
gated in this regard. Some success has been achieved
by photochemically activated chlorine chemistry.*
Since most chlorides of metals are not volatile at low
temperatures, it is believed that the cleaning action is
achieved by the slight etching
of
the silicon substrate
and that the metallic contaminants are "lifted
off'
the
surface of the wafer together with the volatile chlorides
of
silicon. As
in
the case
of
trace organic clean, chloride
chemistries based
on
plasma activation have also been
investigated and have achieved some degree of success.
Alternative nonhalogen-based Chemistries have also
been investigated by using a reactor with sources for
both the deep UV irradiation and the remote microwave
plasma activation.? Gases can be injected into the reac-
tor with or without the
W
or the plasma excitation.
The chemistries are chosen
so
that the reaction prod-
ucts are sufficiently volatile at relatively low tempera-
tures and
so
that the etching of exposed silicon can be
reduced to a minimum. The cleaning efficiencies of
these chemistries have been verified by using trace
amounts of radioactive isotopes of some of the most
commonly encountered contaminant elements (Fe, Cu,
etc.) plated onto bare silicon surfaces.
Single-Wafer Epitaxy
Single-wafer RTCVD technology has been used for
fabrication of in situ-doped homoepitaxial Si and hete-
roepitaxial Si/Ge,Si,, multilayer structures. Epitaxial
Si films have been grown
in
a mixture of DCS, H2, and
the dopant gas at
5
toaf Fig. 82 shows the thickness of
undoped epitaxial films grown
on
heavily doped p'
(100)
wafers versus the DCS flow rate in 200-second
runs
at
=lo00
"C. These data cover a DCS:H2 flow ratio
range
of
14%.
Under these process conditions,
the
deposition takes place in the mass-transport-limited
regime and, as a result, the growth rate and uniformity
are
sensitive to the
DCS
flux
in
the
process chamber. A
wide range
of
controlled growth rates up to more than
1
pm/min can be achieved. Thermal switching of the
process temperature provides a useful capability for
growth
of
multilayer epitaxial structures with fairly
abrupt interfaces.§ Fig. 83 plots the secondary ion mass
*
Reference
105.
t
Reference
49.
$
Reference
49.
5
Reference
84.
spectroscopy
(SIMS)
boron concentration depth profile
for a seven-layer step-boron-doped epitaxial structure
grown following a low-temperature Ge%
+
H2 surface
cleaning (total
of
eight process steps integrated
in
situ).
This structure was grown at
=lo00
"C
on
a p+ substrate
and consists
of
(1)
an undoped epi layer, (2 through
5)
four successively higher step-boron-doped layers,
(6)
an undoped epi layer, and
(7)
a heavily doped layer
at the surface. The
SIMS
profiles were obtained with an
oxygen primary beam under conditions that provided
poor depth resolution. The layer-to-layer transition
width for the top two layers is 280 &decade of dopant
concentration. The actual transition width is smaller
than the observed SIMS value because
of
the SIMS
broadening effects. The transition widths are affected
by the growth temperature and the thermal treatments
during the subsequent growth cycles. Growth of the
epitaxial layers at lower temperatures with remote
microwave plasma excitation should significantly
reduce the transition widths in multilayer epitaxial
structures. Growth of the same structure shown in Fig. 83
without thermal switching (temperature kept at
1000
"C
even during gas switching) results in much wider tran-
sition widths because of the long process gas stabiliza-
tion
times.
Fig. 84 plots the B and Ge SIMS concentration
depth profiles in a seven-layer structure consisting of a
strained p+-Ge,Si,-, layer. This structure was grown
after a GeH,-assisted in situ surface cleaning. The first
five layers are i/p+/i/p+/i silicon regions (i
=
intrinsic),
each grown at
=lo00
"C. The B2H, flow rate during
the growth of the first two p+ layers was ramped in
time, which is one reason for the gradual B concentra-
tion transition between the adjacent i/p' layers. The
sixth and seventh layers are strained p+
-
Ge,Si,, and
n+
Si, respectively. Because of the overall thermal
treatments and
SIMS
broadening, the first intrinsic
layer near the p+ substrate interface cannot be detected
very clearly. The SIMS Ge depth profile indicates a Ge
fraction of
x
=
6%
in the Ge,Si,, layer. The
GeH,:DCS flow ratio during the growth of
this
Ge,Si,,
layer was 2.5 sccm:
100
sccm and the growth was per-
formed at
650-700
"C. The net B2H, flow rate was
about
0.0003
sccm. The
B
concentration depth profile
indicates a Ge,Si,, doping concentration
on
the order
of
1
x
10"
~m-~. Based
on
the SIMS data, the B-to-Ge
concentration ratio in the grown Ge,Si,, film is nearly
equal to the ratio of the number of
B
and Ge atoms
available in the gas phase (or twice the B,H, flow rate
divided by the GeH, flow rate).
Single-Wafer Plasma
Processing
Plasma processes are routinely used
in
semiconduc-
tor fabrication
for
etching of submicron geometries,
deposition and growth of thin films, and in situ organic
and metallic contamination removal. Gas composition,
pressure, and flow rates define the overall chemistry,